How Stress and Chemistry Shape Spiral Patterns—A Surprising Revelation
Unexpected scientific discoveries often lead to groundbreaking insights, and that is exactly what happened when a UCLA researcher stumbled upon intricate spiral patterns formed by a chemical reaction on a germanium wafer.
The experiment, originally designed for a different purpose, revealed how stress and chemistry work together to create unique formations. This finding not only expands the understanding of pattern formation but also connects laboratory experiments to natural biological processes.
Accidental Discovery Leads to a Scientific Breakthrough
While working with metal films on a germanium wafer, UCLA doctoral student Yilin Wong noticed an unexpected change. Small dots appeared on the surface overnight. Out of curiosity, she examined them under a microscope and discovered stunning spiral patterns etched into the wafer. The discovery was entirely unintentional—Wong had initially set up the experiment to explore a different concept related to biomolecule interactions.
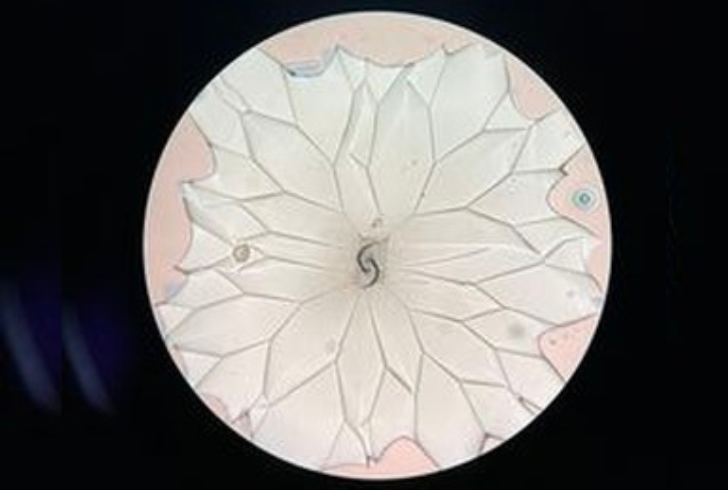
Instagram | uclacollege | An overnight discovery reveals lotus flower patterns on metal wafer.
Her findings led to a deeper investigation. Wong and Professor Giovanni Zocchi examined how these patterns formed and what factors influenced their shapes. They soon realized that tiny modifications in experimental conditions—such as the thickness of the metal film—produced distinct and complex designs. Some patterns resembled Archimedean spirals, while others looked like lotus flowers or radially symmetric structures.
This study, later published in “Physical Review Materials,” showed that chemical reactions alone were not responsible for the formations. Instead, mechanical stress within the metal layers played a crucial role in shaping the patterns.
Understanding the Chemical Process Behind the Patterns
To further investigate, the researchers set up a controlled experiment using:
1. A 10-nanometer layer of chromium
2. A 4-nanometer layer of gold
3. A mild etching solution
After applying the etching solution, they left the wafer in a controlled environment. Over the next 24 to 48 hours, an intriguing process unfolded. The reaction between the metal layers and the solution caused the metal film to delaminate from the germanium surface, creating tension and wrinkles. These wrinkles, combined with catalytic reactions, etched the mesmerizing spiral formations seen under the microscope.
Professor Zocchi described the experiment as essentially creating an electrolytic capacitor, where the chemical and mechanical processes interacted in a self-organizing manner.
The Role of Stress in Pattern Formation
One of the most striking aspects of this discovery is that the patterns are not purely chemical. The study revealed that residual stress in the metal layers played a fundamental role in determining the shapes that emerged.
This means that pre-existing mechanical forces within the material influenced how the chemical reactions unfolded. As a result, the combination of stress and chemistry shaped the final spiral-like and symmetrical structures.
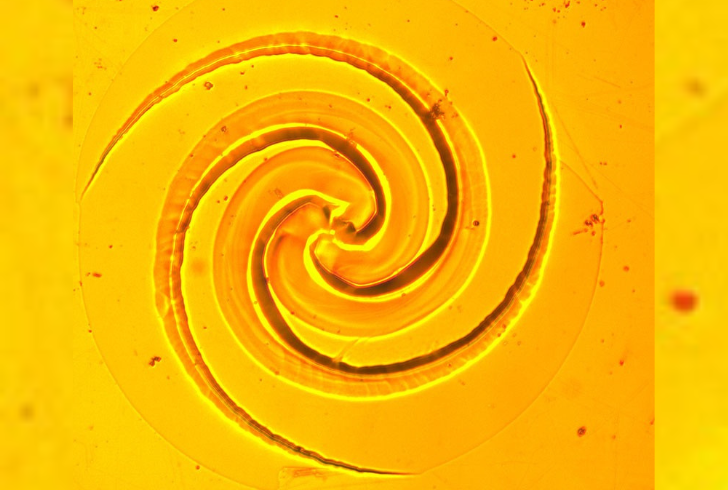
Instagram | uclacollege | Stress and chemistry together create unique patterns overnight.
According to Professor Zocchi, this phenomenon mirrors biological processes where mechanical forces guide growth and development. In nature, enzymes catalyze growth, leading to structural formations in cells and tissues. Wong’s experiment demonstrated a similar effect in a non-living system, making it a valuable model for studying these interactions in a controlled laboratory setting.
Linking Chemical Patterns to Natural Phenomena
The study of pattern formation in chemical reactions dates back to 1951, when Soviet chemist Boris Belousov discovered a chemical system that could oscillate spontaneously. Around the same time, British mathematician Alan Turing developed the reaction-diffusion theory, which explained how chemicals could create complex patterns like stripes or polka dots.
Wong’s findings align with Turing’s theoretical models, providing real-world evidence that chemical and mechanical processes can work together to generate organized patterns.
Advancing the Study of Chemical and Physical Interactions
Although research into chemical pattern formation peaked in the 1980s and 1990s, experimental methods have remained largely unchanged. Most studies have relied on liquid-based reactions, limiting how scientists could observe these patterns.
However, Wong and Zocchi’s system represents a major breakthrough, as it provides a solid-state experimental model to study how chemical reactions and mechanical stress interact. This approach not only expands knowledge in material science but also has potential applications in nanotechnology, bioengineering, and surface chemistry.
Future Implications of the Research
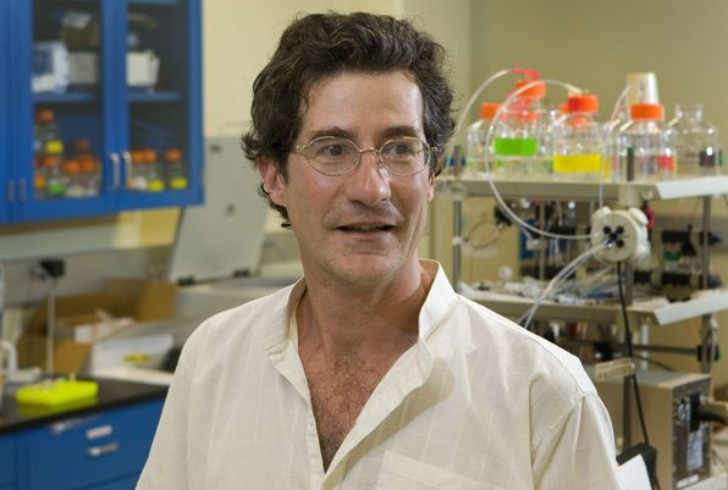
newsroom.ucla.edu | This finding, Zocchi explained, is a precious opportunity to analyze complex, dynamic behaviors in a non-living context.
The discovery raises several exciting possibilities. By refining the experiment, scientists could potentially:
1. Control and design intricate nano-scale patterns for use in microelectronics.
2. Explore how mechanical stress affects biological development, helping researchers understand tissue growth and wound healing.
3. Develop new materials that harness stress-chemistry interactions for advanced engineering applications.
Professor Zocchi emphasized that this discovery offers a rare opportunity to study complex interactions in a non-living but highly dynamic system. This could lead to further breakthroughs in understanding how chemical and mechanical forces shape structures across different scientific fields.
The Future of Pattern Formation
Wong’s discovery highlights how accidental findings often lead to significant scientific progress. What began as a simple mistake turned into an exploration of how stress and chemical reactions create ordered structures.
This research not only expands knowledge of pattern formation but also provides a bridge between material science and biology. By continuing to study these processes, scientists can uncover new ways to manipulate materials, develop advanced nanotechnology, and better understand natural growth mechanisms.
With each experiment, researchers gain deeper insights into the hidden forces shaping the world, proving that even the smallest discoveries can lead to remarkable breakthroughs.